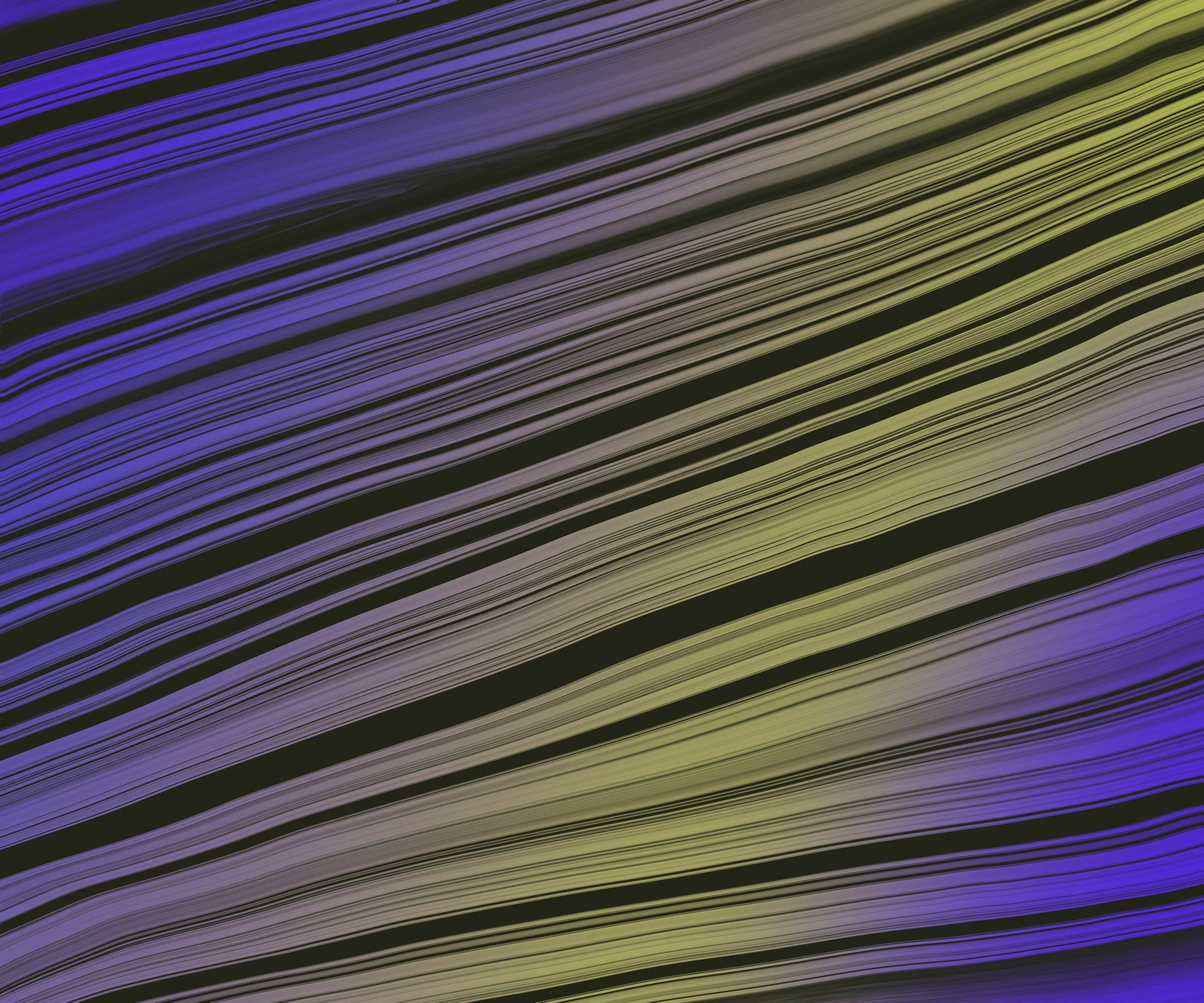
Scientific Illustration
These illustrations were produced by Julian Eskin and Bruce Goode and are made available for download and use under the Creative Commons 4.0 Attribution License. They were made using a combination of Adobe Illustrator, Photoshop, and Blender software.
Fig. 1 Stages of endocytic patch initiation, maturation, and turnover.
The stages of progression (numbered 1–9) of an endocytic patch from initiation to membrane invagination, followed by scission and disassembly/uncoating, make components available for new rounds of patch formation (recycling). Boxes describe the concurrent sequential behavior of the actin cytoskeleton and actin-associated proteins during this process. Each icon represents a group of proteins with related functions, activities, and/or localization.
Goode BL, Eskin JA, Wendland B. Actin and endocytosis in budding yeast. Genetics. 2015;199(2):315-358. doi:10.1534/genetics.112.145540
Goode BL, Eskin JA, Wendland B. Actin and endocytosis in budding yeast. Genetics. 2015;199(2):315-358. doi:10.1534/genetics.112.145540
Fig 2. Endocytic adaptor and coat proteins.
Depicted are the key factors in the three stages of endocytic coat formation, early, middle, and late coat proteins, which are recruited at distinct stages of endocytosis and thus represented as separate layers. Importantly, these represent temporal and not necessarily spatially segregated layers. Lines indicate known binding interactions among components.
Fig 3. A comparison of the four stages in formation and turnover of actin patches vs. cables.
Scheme of the ultrastructure of a cortical actin patch (top) and cable (middle); legend defining the icons (bottom). Both structures are initiated by the nucleation of filaments from ATP-bound G-actin (left). Then, F-actin is stabilized/crosslinked and subsequently severed and disassembled. The released ADP-actin monomers undergo nucleotide exchange to replenish the pool of polymerization-competent ATP-G-actin (right side). Some icons represent groups of proteins with related activities (e.g., Arp2/3 complex NPFs, disassembly cofactors of cofilin). This cycle takes ∼5 sec to complete in each type of actin structure; due to distinct nucleation rates, it occurs within the <300 nm ribosome exclusion zone in an actin patch vs. >5000 nm along the length of an actin cable.
Goode BL, Eskin JA, Wendland B. Actin and endocytosis in budding yeast. Genetics. 2015;199(2):315-358. doi:10.1534/genetics.112.145540
Fig 4. Arp2/3 complex nucleation-promoting factors (NPFs).
The figure depicts three proposed stages of NPF activity. (A) Inhibited NPFs, (B) Network priming, and (C) Active nucleation.
Fig 5. Actin turnover machinery.
Scheme showing the proteins that regulate actin-filament debranching, filament severing and depolymerization, and monomer recycling. Dotted lines are physical interactions. Numbers correspond to the designated steps in actin turnover in which each protein participates.
Goode BL, Eskin JA, Wendland B. Actin and endocytosis in budding yeast. Genetics. 2015;199(2):315-358. doi:10.1534/genetics.112.145540
Fig 6. Structure of Cofilin bound to filament, a model for Twinfilin bound to filament side, and cartoon depictions of free Aip1, Coronin, and Srv2/CAP
Fig 7. (A) Schematic representation of four mechanisms of actin filament nucleation.
Spontaneous assembly of actin monomers (G-actin) into filaments (F-actin) is kinetically unfavorable, however, actin tetramers are stable, and once formed, new subunits will readily associate with the barbed (+) end.
(B) Schematic representation of the two known mechanisms for promoting actin filament elongation.
Formins processively associate with the growing barbed ends of filaments and accelerate elongation by recruiting profilin-bound actin monomers and delivering the, to the barbed end.